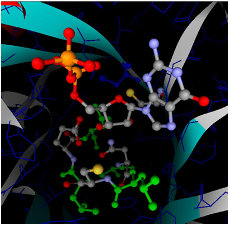
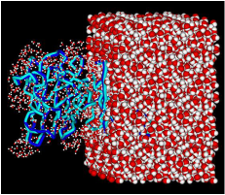
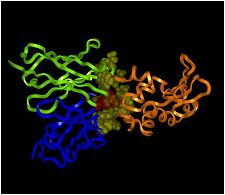
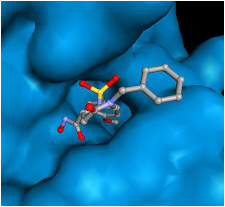
Research Areas
Enzyme Catalysis:
We
have been performing the study of catalytic and inhibition mechanisms
of several enzymes, such as Class I
Ribonucleotide Reductase, Pyruvate Formate Lyase, Farnesyltransferase,
Fumarate Reductase, Cox-2, Uroporphyrinogen III Decarboxylase,
Glutathione Transferase, Superoxide Dismutase, Thioredoxin, HIV-1
Protease, Reverse
Transcriptase and Integrase, PLP-dependent enzymes, HMG-CoA-Reductase,
Asparaginase, Renin, ACE-II and several glucosidades, among others. We
have been using QM/MM methodologies with the QM part described at the
DFT
level with large basis sets, embedded in complete enzyme models
described at the MM level.
Molecular Dynamics of Proteins:
We
have been addressing many aspects of
enzyme catalysis via molecular dynamics simulations. These include
conformational changes, detection of water channels and water hydration
sites, evaluation of protein flexibility, or refinement of
enzyme:substrate complexes obtained from docking/modelling studies. Cu,
Zn Superoxide Dismutase, Farnesyltransferase, Gluthatione Transferase
or HIV-1 Reverse Transcriptase are some of the studied systems.
Parameter Development is also an area in which we have been working on, e.g. we have developed molecular mechanics parameters for metal
enzymes in several ligand environments. The parameters have been
obtained by fitting to DFT potential energy surfaces and are committed
to the Amber force field. Parameters for
biological membranes have also been developed.
Computational Mutagenesis:
Protein
complexation regulates a large number of cellular events, and to
interfere with protein:protein complexes is of the utmost therapeutic
importance. Alanine scanning mutagenesis of protein-protein interfacial
residues is currently performed to detect the hot spots for protein
complexation. These are the regions that must be drug-targeted.
We have developed a computational protocol, based on MM-PBSA
calculations, that predicts differences in binding free energies
between the wild-type and alanine mutated complexes with an average
unsigned error of 0.80 kcal/mol, and a maximum error of 2.5 kcal/mol.
It was benchmarked with a set of 46 mutations, and permits a systematic
scanning mutagenesis of protein-protein interfaces. We have recently
shown that the method is as accurate as TI but at a fraction of the
computational cost.
Drug Transport Across Cell Membranes:
In addition to therapeutic effect, drugs need to exhibit favourable
absorption, distribution, metabolism and excretion (ADME)
characteristics to produce a desirable response in vivo.
For absorption and tissue distribution, a drug must be absorbed through
a succession of lipid bilayers before reaching its target. This makes
cell membrane permeation of paramount importance, and a clear
understanding of this process is crucial for rational drug design.
Drug Discovery:
Drug
discovery is an area in which we have been working on. Basically, we have
been exploring ways of optimizing lead compounds. Usually we start from
a structure of a receptor and try to find hit compounds that bind the
receptor with virtual screening techniques. After experimental
validation, we try to perform subtle
modifications in the drug which improve its affinity (calculated with FEP/TI techniques) without
compromising its ADME properties.